The evolution towards smart grids: a new emerging framework
in cooperation with CESI - by Bruno Cova
The electricity sector is undergoing a profound disruption that will affect its entire value chain over the coming decades. New resources are connecting to the grid, such as distributed storage and electric vehicles, while renewable generation is spreading, providing a local and emission-free alternative to the conventional power plants. Demand flips its role from a random energy consumer into a component that modifies the intake of energy according to the needs of the power system.
Within this context, the electricity networks also need to evolve in order to support the innovative solutions implemented in the system, with several technologies supporting this process: Smart metering is considered one of the main enablers, whereas communications systems, data collection and analytics represent the backbone for the exchange and analysis of information: paramount functionalities for a secure operation of the entire system.

Therefore, we are witnessing a shift from the traditional power networks to the so-called “smart grid”, characterized by real-time monitoring and control of the various distributed energy resources connected, implying a bidirectional energy flow, which must be properly handled to ensure a reliable and secure electric system.
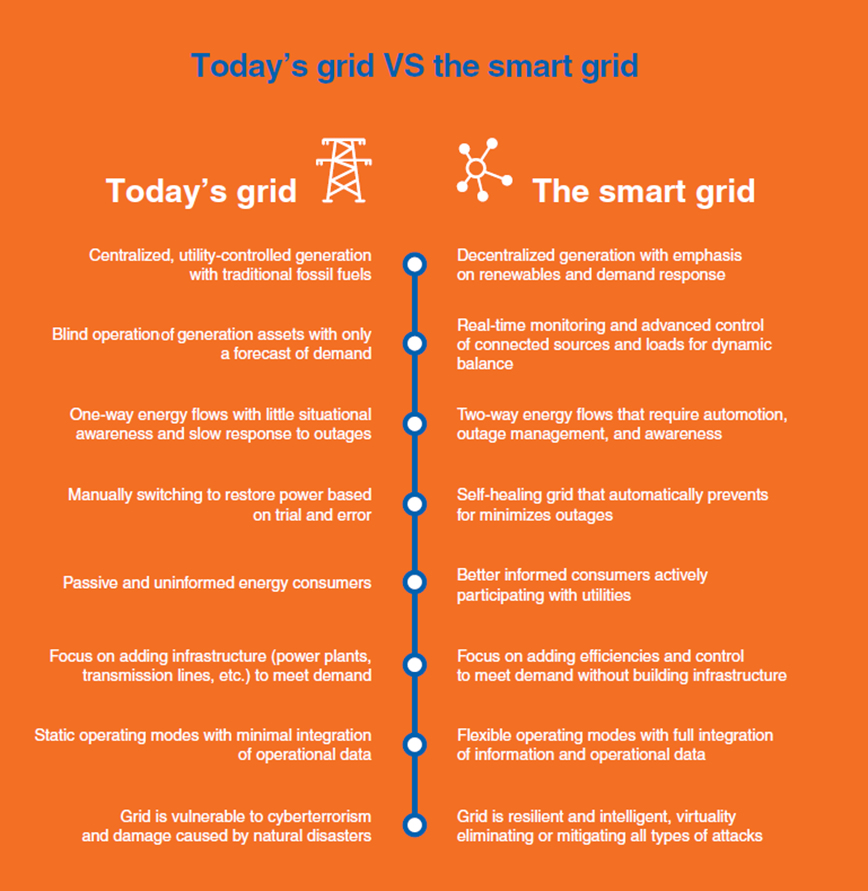
The smart grid could be represented in four different domains, as shown in Figure 3:
- Grid Physical Asset Layer: This is the foundation of the smart grid, since it includes all the systems that collect measures and implement actions to manage the energy flows. It includes smart metering infrastructure, diagnostic sensors, switching gears and voltage and VAR control[1].
- Grid Network and Control Layer: The signals coming from the physical domain are processed and transmitted here, with the primary function of data validation and of establishing connections between several points of the network. Data acquisition interfaces and communication protocols belong to this domain.
- Grid Application Layer: In this domain, the signals are elaborated within advanced algorithms to realize different functionalities. It includes the automated management systems of distribution networks, the dispatching of distributed energy resources and demand management.
- Soft Grid Layer: The data are aggregated and transformed into high-level information for strategic insights and predictive functionalities, enabling optimized network operations.
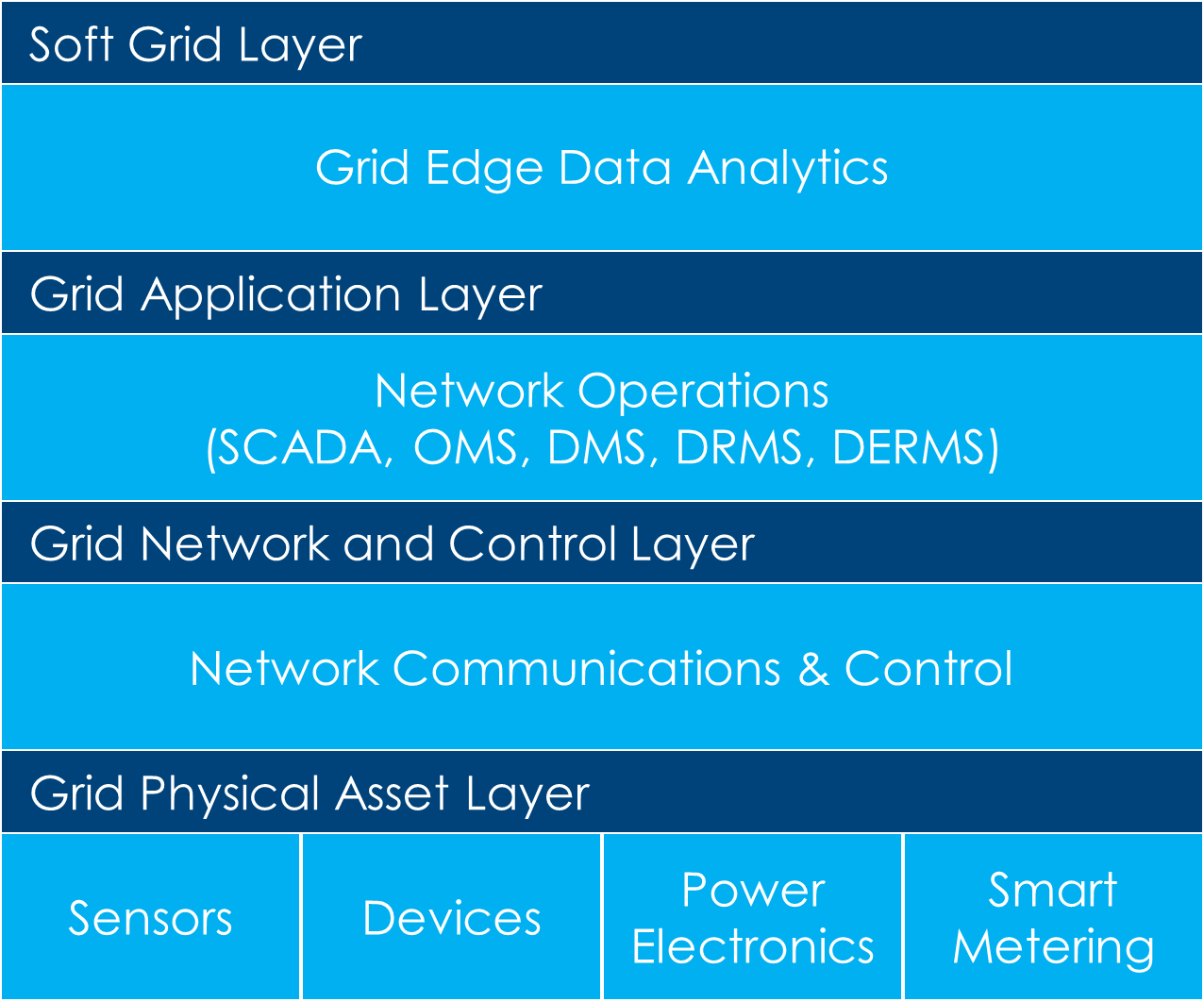
This transformation certainly entails massive investments for the design, development and implementation of the solutions needed to make the grid “smarter”. There has been a high level of such investments for several years now, even if recent spending has decreased, as displayed in Figure 4, with a 7% reduction in 2019 compared to the previous year. In parallel, the digitalization of the grid continues to show a positive trend, with a constant increase over the years.
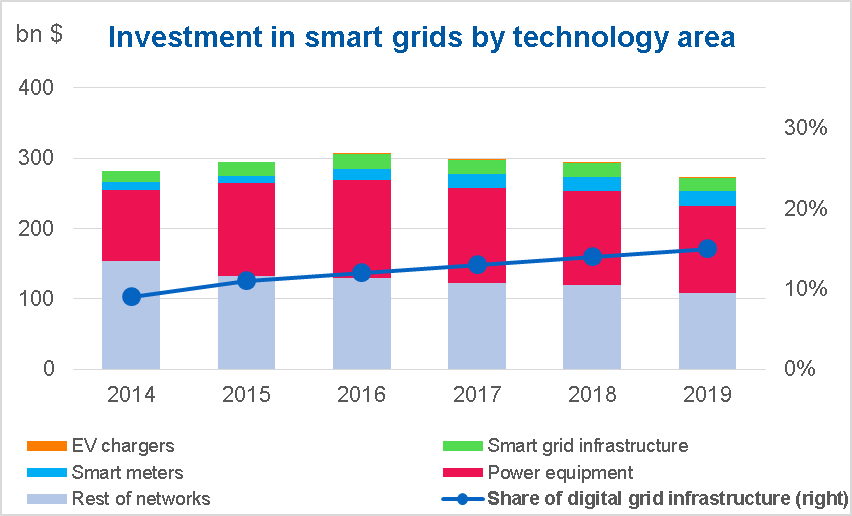
In the future, the level of investments in power networks is required to grow significantly: According to the IEA report “Net Zero by 2050”, the agency’s roadmap towards this goal, grid investments will triple by 2030 reaching $820 billion and achieve the highest value in 2040 with $1 trillion, as presented in Figure 5.
Just to give an order of magnitude, IEA indicates that global electricity network length will double by 2040, implying a capillary deployment of power infrastructures worldwide. More than half of the investments will be required to accommodate the new demand, while the rest will be split between renewables integration and the replacement of old infrastructure.
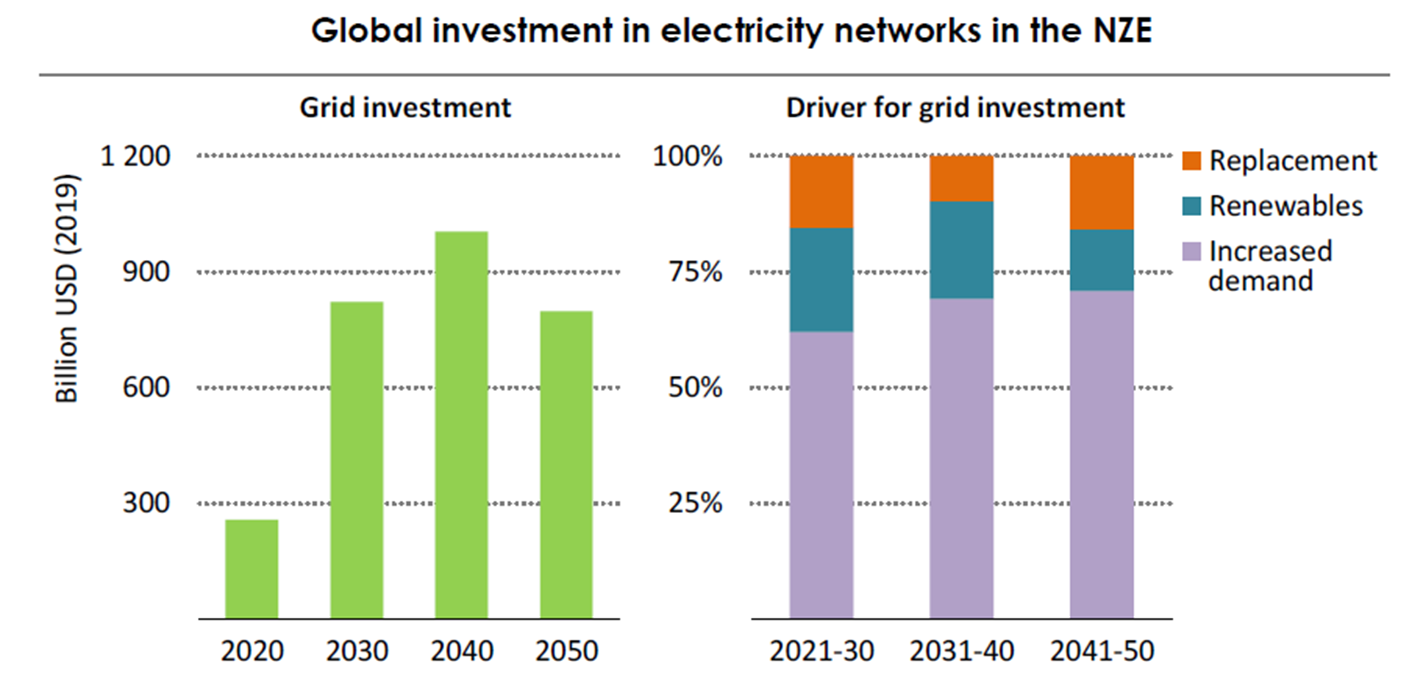
Indeed, the need for a renewal of the electricity network is due to the ageing of power lines: According to research developed by Deloitte and Eurelectric (Figure 6), about 70% of low voltage (LV) power lines in 2020 were over 20 years old. Replacement is also necessary to incorporate digital functions within the grid, which as mentioned above will drive the process of transition towards smart grids.
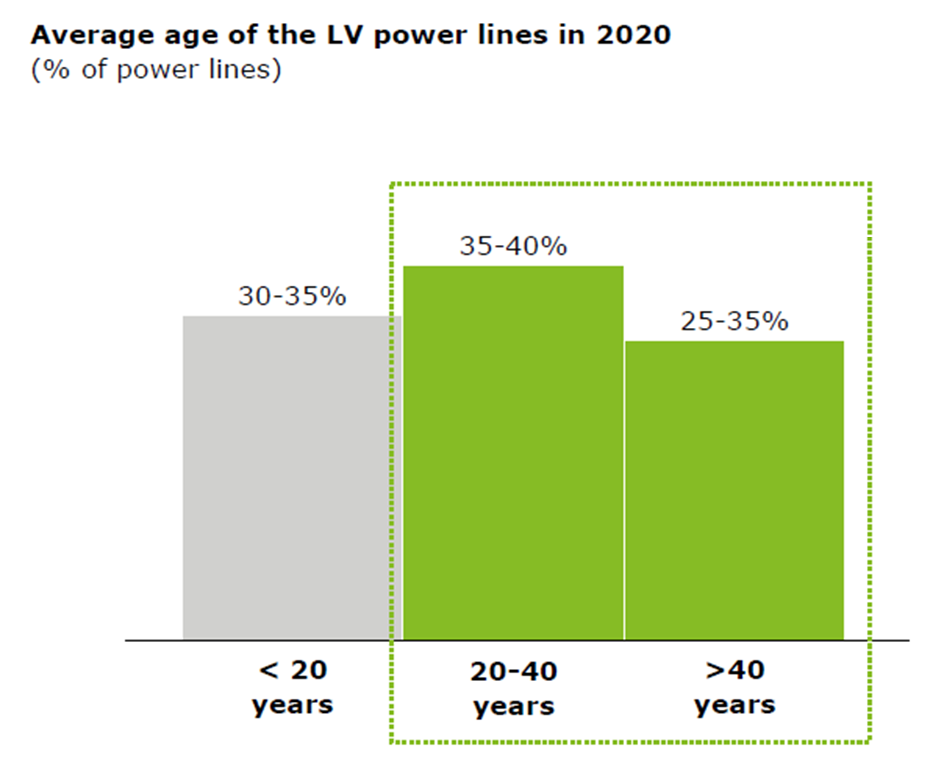
The pivotal role of digitalization is also highlighted by the market size of grid software, which gives indications on the fields where utilities are focusing efforts. As seen in Figure 7, in 2020 the market size of grid software was $1.5 billion, in which Advanced Distribution Management Systems (ADMS) represent the most relevant application due to their importance in handling and optimizing a complete set of functionalities for the correct operation of the distribution networks.
These are followed by asset performance management, which enables a better reliability of the grid and an increase in the lifespan of power equipment. Within five years, the market size of smart grid software will grow by 60%, amounting to $2.4 billion in 2025: Also in this case, ADMS and asset performance management are the main categories, while the segments with the highest growth rate are drone software and augmented reality to improve maintenance and inspections operations. The last category is represented by Distributed Energy Resource Management Systems (DERMS), since the critical mass of Distributed energy resources (DER) to imply an integrated control would be achieved only after 2025.
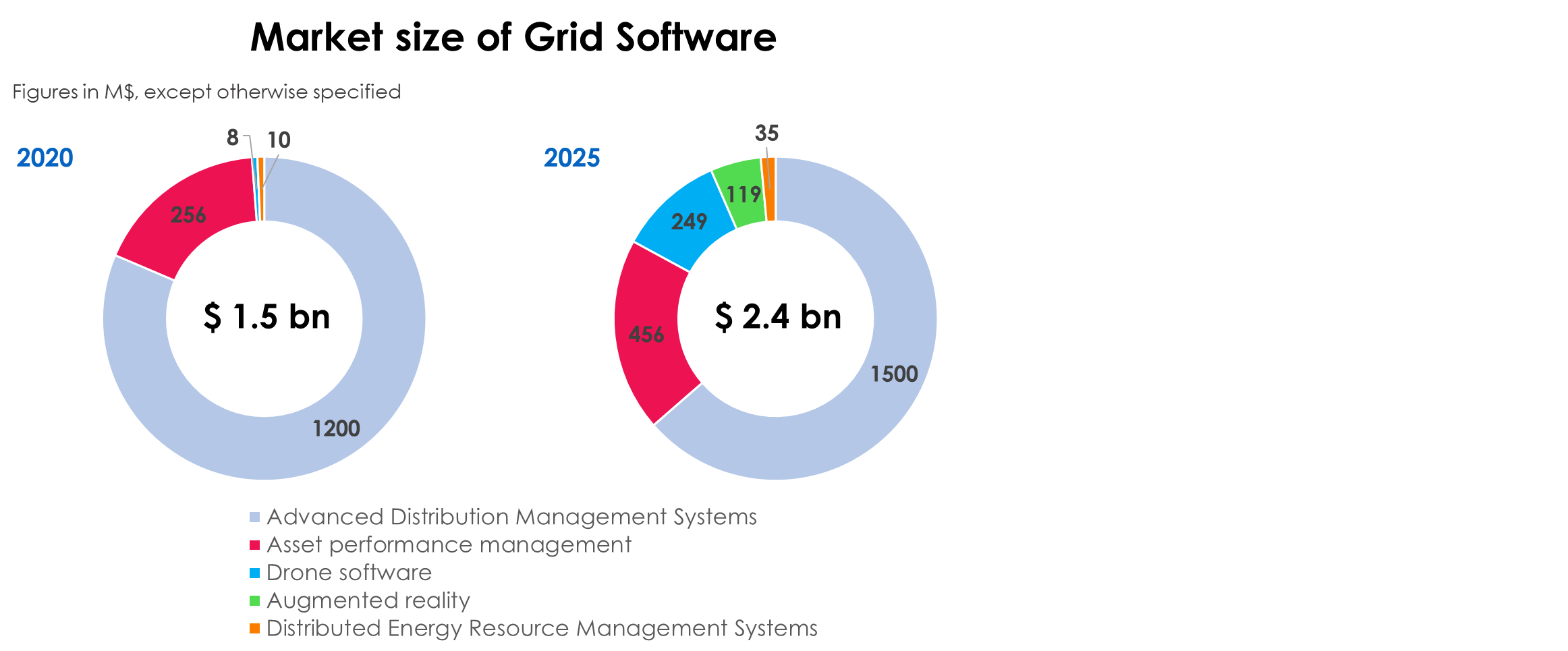
A relevant technology allowing an optimized management of the grid is given by smart meters, which collect a remarkable amount of data and provide advanced functionalities for the benefit of the grid and the final customer. However, the installation rate of smart meters is not uniform in the world.
As displayed in Figure 8, at the end of 2020 in Europe there were several countries in the second wave rollout, while others reached only 10% or fewer customers, with an overall 45% penetration rate.
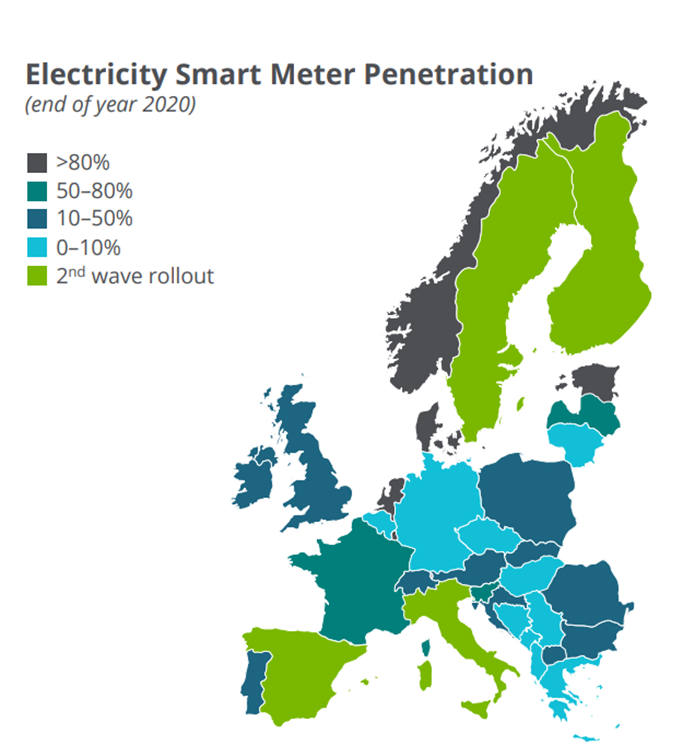
This uneven distribution is also present in the US, as shown in Figure 9, with few states having less than a 15% installation rate, several northern and central states with a deployment between 15% and 50% and the rest with a penetration rate above 50%.

The transformation of the electricity sector towards the smart grid dimension does not only regard technology, but has a notable impact on the underlying economic model, which follows the same direction from a centralized asset-intensive approach towards distributed, digitalized and shared resources. Within this context, the energy-as-a-service business model is emerging, focused on the shift from trading kWh to selling services to customers: an end-to-end management of assets and resources through a recurring subscription fee or a performance-based contract with a profit/saving sharing mechanism. In this way the final user does not incur in expensive investments and is provided with an all-in-one hassle-free package for demand management, distributed generation and storage or energy efficiency while targeting savings, sustainability and system flexibility. The International Renewable Energy Agency (IRENA) groups the services supplied by the model in three categories:
- Energy advice, providing suggestions and formulating strategies suited to customers through the collection and analysis of a large amount of data
- Energy assets installation, with a turnkey solution for renewable systems, energy storage, microgrids or EV charging infrastructure
- Energy management, providing monitoring, control and optimization of energy generation and consumption, with the possibility to improve the profitability through energy market participation
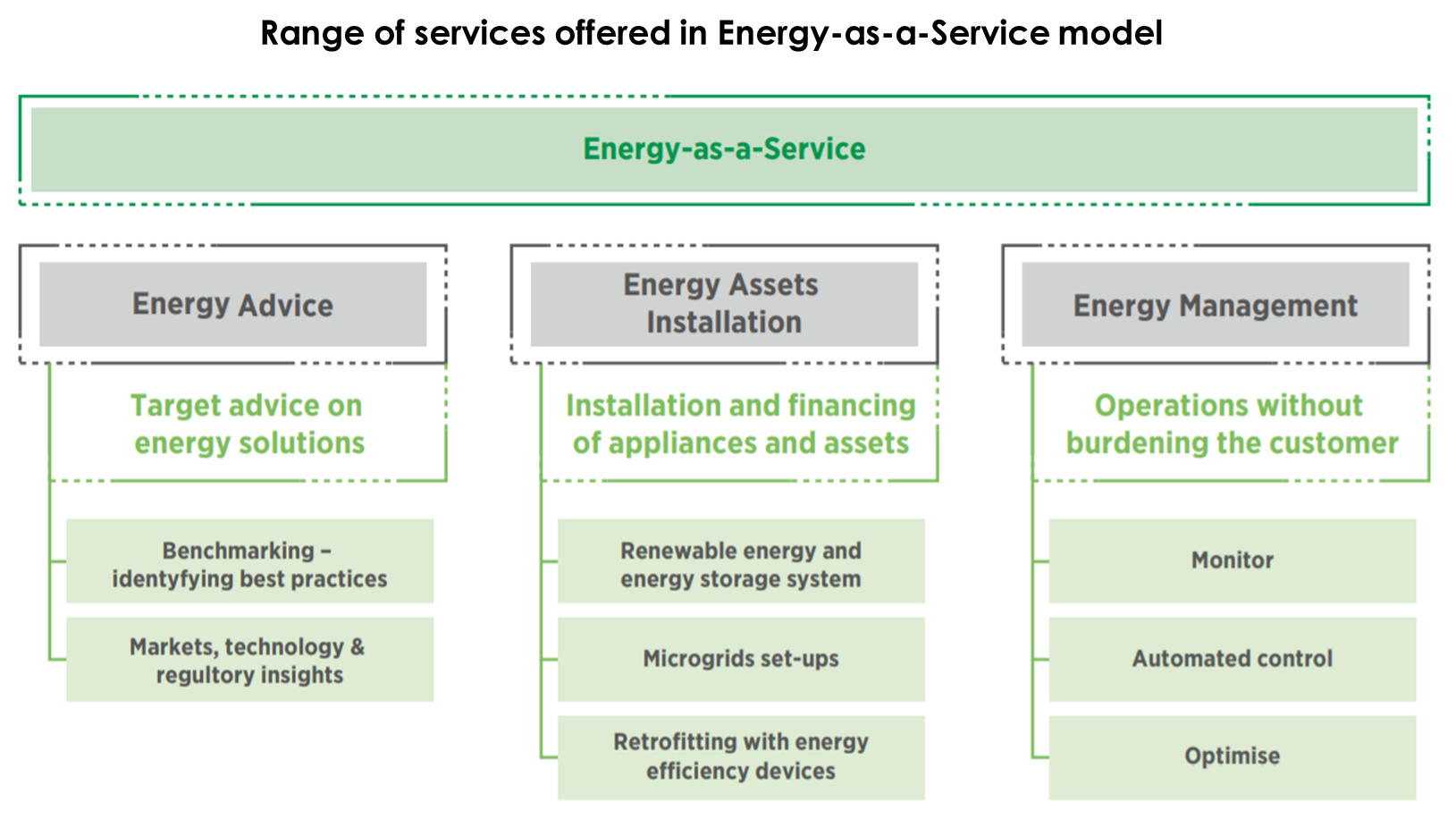
The general framework of the energy-as-a-service model is displayed in Figure 11, where the provider is the interface with several stakeholders. The provider works with the customer to design and implement the project in exchange for an economic return. On the other side, there is coordination with third-party vendors for the realization of the solution and there could eventually be an interaction with the investor (sometimes coinciding with the provider), which provides the necessary financing for the project.
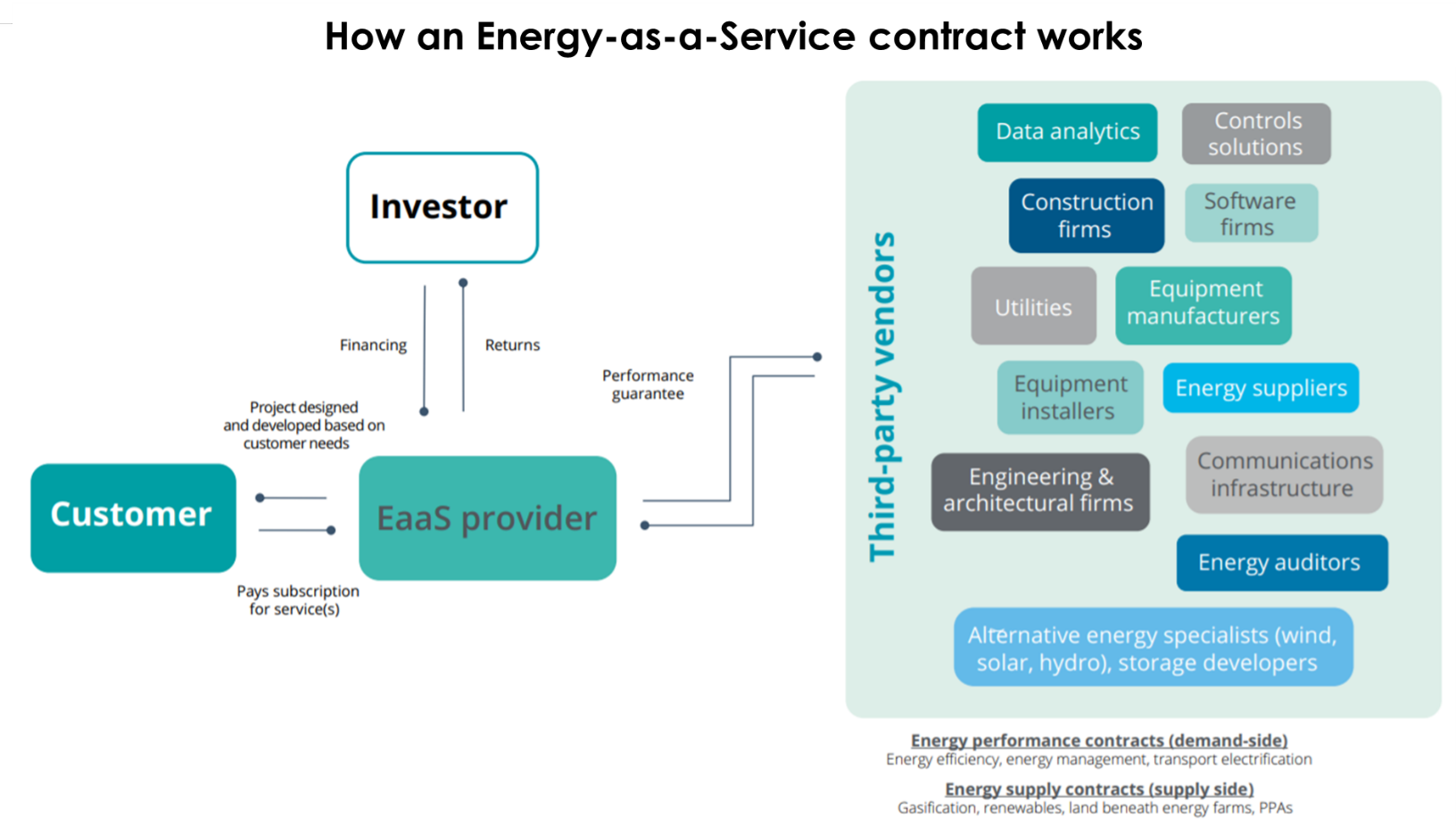
The road towards the realization of smart grids is still long, but it represents a critical factor in the positive outcome of the energy transition without compromising the quality and the continuity of such an essential service.
Footnotes:
[1] Volt-VAR Control or VVC refers to the process of managing voltage levels and reactive power (VAR) throughout the power distribution systems.”
Smart grids for the energy transition – in cooperation with CESI