Ensuring energy security in a carbon-free economy
in cooperation with CESI - by Bruno Cova and Luca Migliorini
In the era of decarbonization, ensuring security of supply shall remain one of the key pillars in the energy systems, particularly in the power systems, where energy storage is still a challenge. Two energy security dimensions shall be addressed to identify coherent solutions for a robust energy system:
- vulnerabilities against internal events;
- vulnerabilities against external shocks;
as conceptually shown in Figure 1 referring to the European gas and power systems.
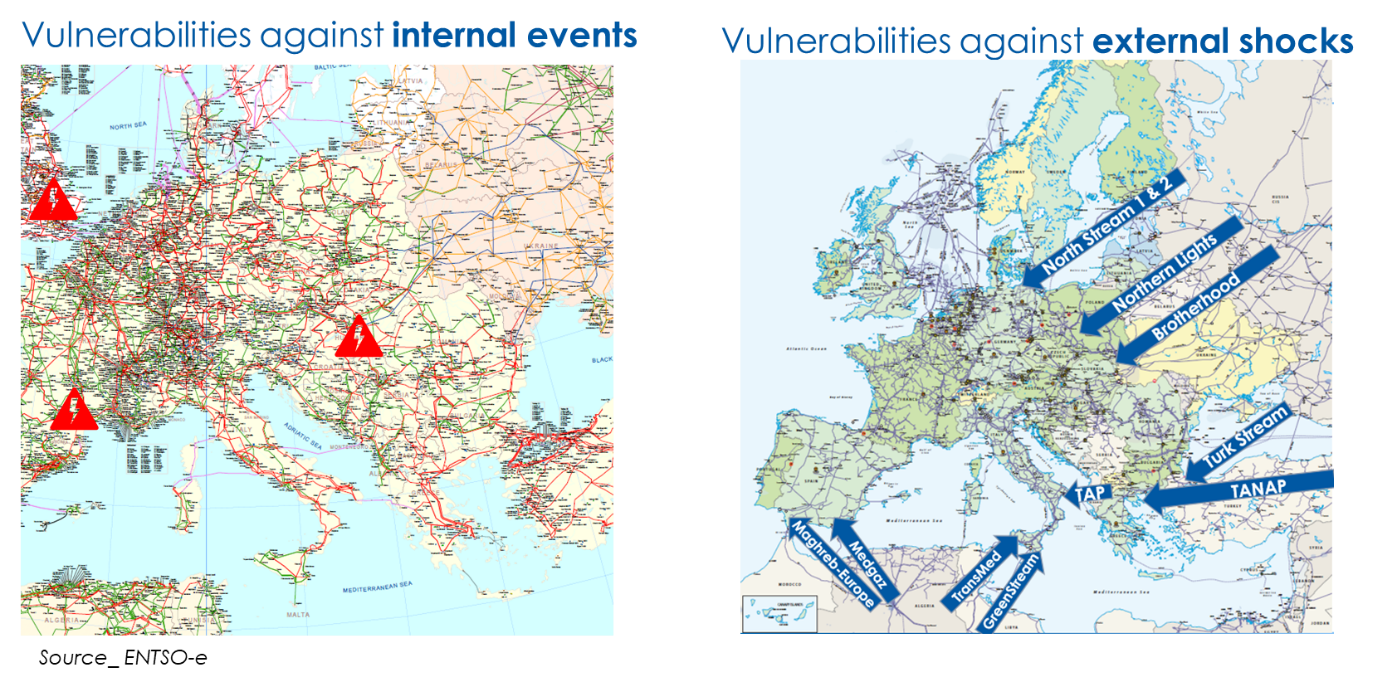
Vulnerability against internal events refer to the ability of an energy system to withstand credible faults without any interruption of energy supply (the so-called N-m security criterion) and to react in a controlled way in case of faults exceeding the adopted security criteria (controlled load shedding). Some signals on the progressive fragilization of the European power system have already emerged: only in 2021 two events caused the splitting of the interconnected system in two separate “islands” (January 8th and July 24th).
In other cases, frequency drops exceeding 100ms were recorded, basically caused by errors in forecasting wind production. These phenomena can be solved by strengthening the interconnections and enhancing the flexibility of the system in order to cope with the steepest ramps up or down caused by variable renewable energy sources (RES).
Concerning external vulnerabilities, enhancing the exploitation of local RES has a positive effect. For instance, Europe as a whole is currently importing 58% of its energy demand, making it the largest net energy importer among the G20 members. Ninety-five percent of oil products are imported, while for natural gas it is 83%, with eleven EU member states depending on a single external supplier for more than 75% and 17 member states importing more than 90% of their natural gas+ demand. All this makes the system too vulnerable to supply shocks. A relatively tiny incident occurred on December 12, 2017 at the Baumgarten gas hub in Austria causing a widespread gas disruption across Europe with the gas spot price rocketing to €51/MWh, meaning a 120% hike compared to the day before (€23.4/MWh). More recently we have witnessed the adverse impact in Europe of the natural gas rally with prices soaring almost seven-fold compared to one year ago and a consequent impact on the power markets, especially in the countries heavily relying on natural gas for power generation, like Italy. This surge in energy prices risks slowing down the post-pandemic economic recovery.
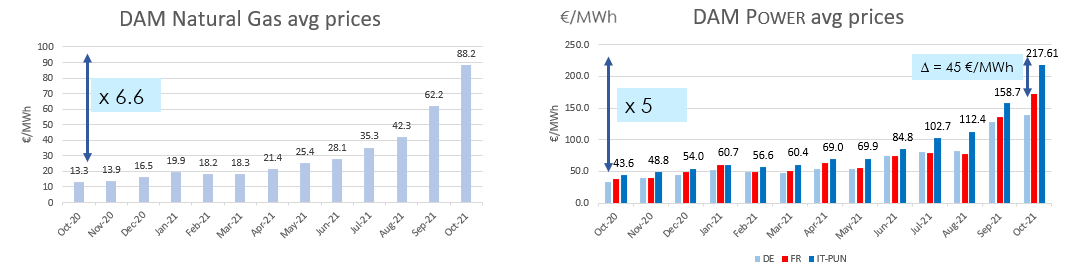
There is a widespread consensus that a higher reliance on renewable energy sources would make Europe stronger against possible external shocks; indeed, according to the global measurement technology leading company Vaisala the potential of renewable resources in Europe is very significant, especially thanks to wind availability in Northern Europe and the solar abundance in Southern Europe, overcompensating the level of oil and gas reserves currently present in the continent. Therefore, with decarbonization Europe will likely reduce its energy dependency on foreign countries.
Moving to new technologies based on new materials
The (r)evolution to a carbon-free economy entails the adoption of new technologies in all sectors, notably:
- power generation: wind and solar;
- road transport (e-mobility): batteries, fuel cells and electric traction motors;
- hard to abate sectors: “green”, or “low carbon”, hydrogen generated through electrolysis.
Focusing on wind and solar power generation and e-mobility, we can see that the new technologies are heavily relying on new materials, as displayed in Figure 3. “Green” hydrogen is in turn dependent on wind and solar technologies, since electrolyzers must be supplied with renewal energy source electricity. Furthermore, the modern technology of electrolyzers based on Proton Exchange Membrane typically use noble metals such as Pt/Pd-based catalysts as cathode for the hydrogen reaction and RuO2/IrO2 catalysts as anode for oxygen reaction.
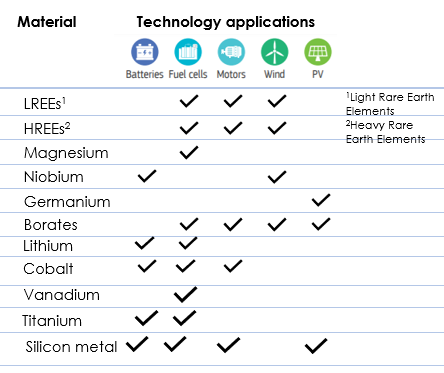
The progress towards a carbon free economy will entail a massive use of new categories of materials. According to EU estimates, “for electric vehicle batteries and energy storage, the EU would need up to 18 times more lithium and 5 times more cobalt in 2030, and almost 60 times more lithium and 15 times more cobalt in 2050, compared to the current supply to the whole EU economy. Demand for rare earth used in permanent magnets could increase tenfold by 2050”.[1]
In other words, we are evolving from an economy based on steel, copper and aluminum to an economy based on a much wider set of materials. This evolution sets daunting challenges related to availability and supply chain.
Availability of some raw materials is a key concern. Let us take the case of e-mobility. There are currently more than one billion vehicles worldwide. At a global scale, almost all countries are gearing up to rapidly shift from internal combustion engines to electric vehicles. China is targeting a 15% share of electric vehicles by 2025, rising quickly to 40% in 2030. Other countries in Europe have set deadlines to end the sale of gasoline and diesel cars: Germany in 2030 and France and UK in 2040, while the European Commission is proposing 2035. By 2030 estimations vary between 100 million (Bloomberg) and 200 million (Barclays) of electric vehicles on the roads with an intermediate scenario targeting 170 million.
Read also: An outlook on the green transition after COP26
The current technologies for car batteries are based on Lithium-ion (Li-ion) or nickel-metal hydride (NiMH), containing lithium and cobalt. Both materials are expected to face a vertiginous rise in their consumption. Whereas resources of lithium are relatively abundant, though concentrated in few countries like Australia, Chile and Bolivia, cobalt is in a much shorter supply. According to the US Geological Survey, cobalt world reserves that can be economically exploited with today’s technologies are estimated in about seven million tonnes, which represents only 57 years of production referred to the pre-pandemic level. The booming of electric vehicles with Li-ion or NiMH batteries will exhaust cobalt reserves in a much shorter time. Furthermore, one shall consider the competition between different industrial sectors using cobalt, which is adopted for the superalloys, steel manufacturing and medicine sectors. Thus, only about 50% of the current cobalt production could be used for rechargeable batteries. Similar considerations apply to other raw materials.
Supply chain of components needed for the new technologies is another concern. In fact, many raw minerals are extracted and processed from one or two key countries creating an even bigger vulnerability for the importing countries, notably the OECD countries. Considering the EU, we can see (Figure 4) an almost full dependence on key materials from external countries.
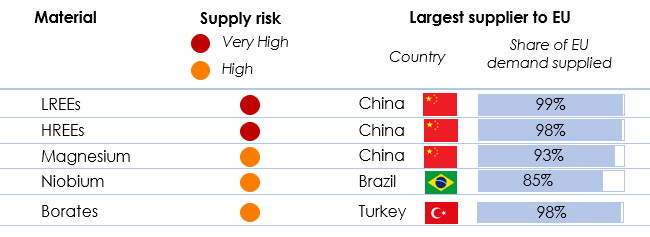
A clear sign of this vulnerability emerged in 2010 when, following a dispute over fishing matters between Japan and China, the latter cut exports of vital rare earth materials. The restriction on rare earths exports had an immediate impact on their market prices, which soared from $16/kg in Q2 2010 to nearly $60/kg in October 2010. In the following years, after lifting restrictions on rare earths exports, China kept prices low, discouraging possible competitors to undertake heavy upfront investments on the extraction and processing of rare earth minerals.
The example above was an early warning of the forthcoming new vulnerabilities when moving to a massive implementation of the new technologies necessary for renewable power generation, e-mobility and many other sectors like robotics and defense. Consequently, in September 2020 the EU issued an updated list of critical raw materials. Out of 83 materials screened in their whole value chain from extraction to processing as many as 30 materials were identified as critical – this was more than twofold the critical materials (14) identified in 2011. In general, for most materials the EU is already now reliant on between 75% and 100% from imports and following the current trend the situation will be rapidly worsening in the coming years considering also the competition from other OECD and emerging countries in importing raw materials.
Measures to mitigate risks
Constraints that are emerging in the supply chain following the worldwide post-pandemic recovery must be addressed and solved now. Measures to mitigate risks in supply chain of raw materials can be clustered in the following four categories:
Regarding recycling in Europe, materials used for many decades in the industry like iron, zinc, platinum are more than 50% recycled, giving a good contribution to the circular economy. On the contrary, most of materials used in the green economy are poorly or not recycled at all. There are two main obstacles to be addressed, such as information on the materials contained in the dismissed products and the availability of efficient technologies to reprocess the products and extract the materials for their reuse.
The first obstacle can be overcome by clearly labelling each product with its material contents as happens with the food industry. This is already happening to some extent with old lamps using tungsten that is recycled in a quite good share: 42% of the EU demand is covered by recycled tungsten.
Technologies to reprocess the products are still in many cases either energy intensive and inefficient or economically not viable. For instance, the recycling of lithium contained in electric vehicle batteries costs more than purchasing new lithium. Research programs have been launched by the European Commission to identify practical solutions for material recycling. In parallel, some industrial initiatives for the recycling of batteries have already been undertaken in Europe (Umicore-Belgium, Northvolt-Sweden) and the US (Tesla Gigafactory). But as we await more mature recycling technologies, an appealing solution consists in the reuse. For example, when a car battery reaches the end of life for mobility, it can still be efficiently used in the power sector for levelling the volatile wind and solar production.
Substitution is a further action to mitigate risks. Some rare earths are used for their permanent magnetic characteristics (for example, neodymium, samarium, dysprosium) in electric traction motors and wind turbine rotors. There are alternative solutions to avoid using such critical materials. In e-mobility, BWM has launched the iX model with a brushed electric motor not requiring permanent magnets. Other car manufactures (Mercedes) are working to adopt batteries with a low percentage of cobalt, though with a higher share of nickel, with the long-term objective of substituting this critical material. Similarly, the use of asynchronous induction generators with cage rotors in the wind generators (for example, Vestas) is an alternative to permanent magnets. The current volatility of raw materials prices is a driver for the industry to adopt technological solutions based on materials more widely available.
Regarding new greener technologies, the carbon footprint and, in general, the environmental impact of extracting and processing raw materials needed in the green economy are two other issues to be addressed. The extraction and processing of rare earths requires a complex sequence of chemical treatments with an adverse impact on the land and waters. Furthermore, most of the raw minerals containing rare earths (for example, monazite) also include thorium, a radioactive element that becomes concentrated in the wastes. Finally, given their low contents per volume of raw materials, the production of 1kg of rare earth oxide entails between one and two tons of waste material.
This heavy impact on the territory, in turn, implies enormous costs for remediation. China Water Risk (a non-profit initiative based in Hong Kong dedicated to highlighting and addressing business and environmental risk arising from the country’s water crisis) estimated in 2016 that the restoration of the mining area in the Ganzhou district would cost $6 billion and the worrying aspect is that the Jangxi province, where the Ganzhou district is located, counts only for 8.6% of the Chinese rare earths production.
Read also: Which roadmap for energy transition?
A further environmental impact is on greenhouse gas emissions. Let us consider lithium, one of the less complex materials to be extracted and processed. Hard rock mining to extract the mineral and then roast it using fossil fuels leaves scars in the landscape, requires a large amount of water and releases 15 tons of CO2 for every tonne of lithium. In most favorable cases, where lithium is collected from salt lakes in South America the carbon footprint drops to five tonnes of CO2. Considering the expected dramatic increase of battery powered electric vehicles, the global lithium demand would attain about 450kton in 2030 implying 4.5 million tons of CO2 emissions.[2]. Other materials have much more impact on greenhouse gas emissions. Thus, the adoption of new technologies will play a key role. Lithium can be obtained from a geothermal steam with limited or no impact on the environment: “green lithium”. The groundwater in sedimentary basins of the Upper Rhine in Germany is particularly rich in lithium with a concentration of about 150mg/liter of water. The geothermal plant in Bruchsal (Germany) could produce green lithium sufficient for 20,000 electric batteries. A wider exploitation of the Rhine Basin could supply ten times more “green lithium”. Recent sampling highlighted that there are many sedimentary basins rich in lithium and some of them are in Europe, for example a promising area is the Po valley in Italy.
Concerning domestic production, sourcing raw materials directly from Europe is a further measure to mitigate vulnerability of the carbon-free economy. The possibility of producing lithium from geothermal basins in Europe is one example, but indeed the continent is rich in many other raw materials as highlighted by EuroGeoSurveys (Figure 5). The European Battery Alliance launched in 2017 has namely the aim of making Europe independent in the production of batteries by 2025. A key aspect to insource production of raw materials is the social acceptance of mining activities. Some areas still relying on coal mining can shift their production to critical raw materials supported by appropriate funding (Just Transition Mechanism) with a positive social impact.
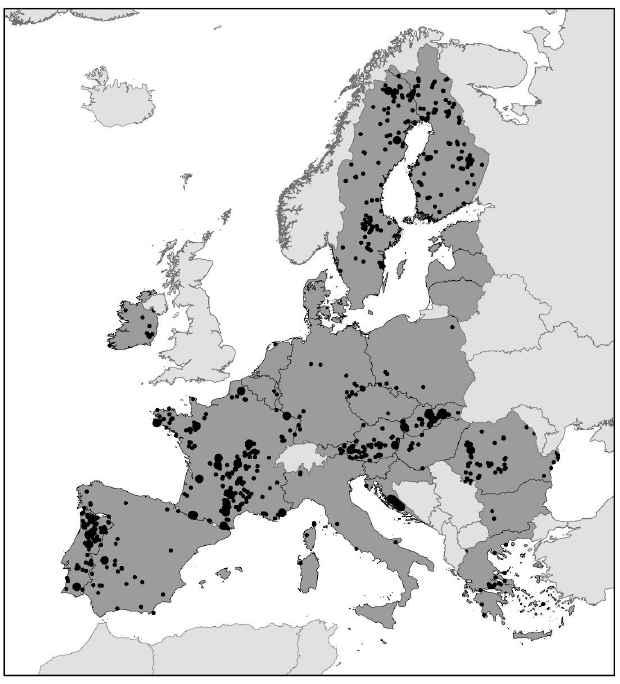
The need for new emissions indicators
The (r)evolution to a carbon-free economy is a highly complex process related not only to a shift from fossil to renewable energies for the generation of energy carriers (electricity and hydrogen), but to the technologies and the chains to produce the various high-tech components. A clear view of the whole supply chain for all materials is a key step in addressing and solving the already present vulnerabilities.
Furthermore, the decarbonization pathway in the OECD countries must not shift adverse environmental impacts and greenhouse gas emissions to supplier countries.
Thus, when elaborating scenarios targeting greenhouse gas emission cuts, one should consider a new set of indicators related to the emissions during the whole supply chain of components until their dismantling. Appropriate labelling of the materials contained in components used in the green economy would allow to have a better awareness of their carbon footprint and risks in supply chain leading consequently to the adoption of mitigation measures, as outline above.
These indicators will be added to the traditional ones related to the economic indexes of the various projects, the technical performances of energy systems, and the compliance of the various development plans with the UN Sustainable Development Goals.
Footnotes:
[1] EC communication on “Critical Raw Materials Resilience: Charting a Path towards greater Security and Sustainability”, Sept. 2020
[2] Assuming an average carbon emission of 10 kgCO2/kgLi
The green transition and energy security after COP26 – in cooperation with CESI